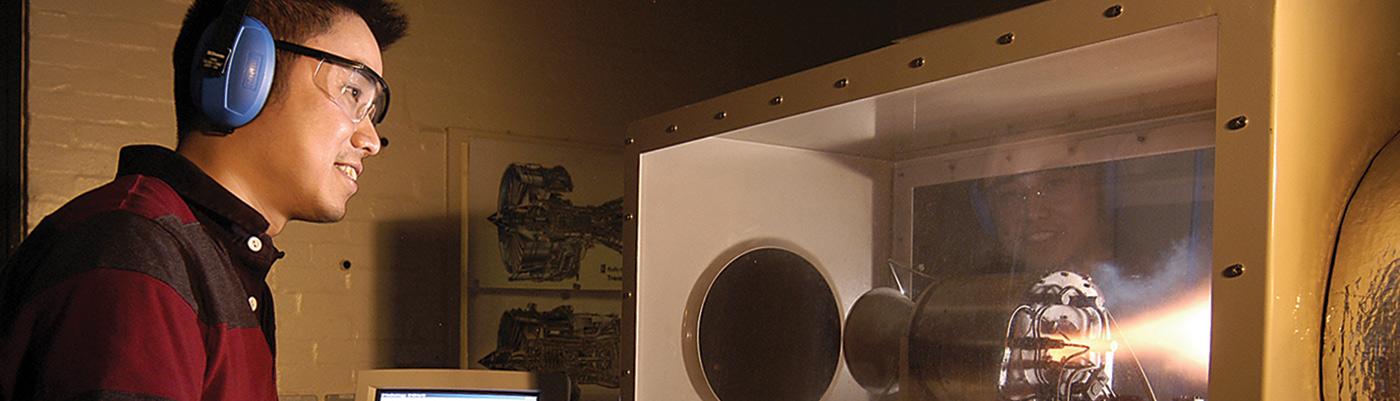
Our research
Our academically excellent research advances engineering knowledge and addresses major challenges of industrial and societal importance.
We collaborate with leading academics, institutions and organisations internationally to inspire and advance our research.
We maintain expertise across engineering disciplines and engage with users of research from academics to industrial and policy stakeholders to frame our research agenda.
For society to reap the benefits of engineering research, we establish multidisciplinary collaborations, drawing together relevant expertise from across the academic spectrum.
Explore our research themes, the centres we work with, and much more below.
Themes
Our research spans six linked themes, with a strong focus on enabling positive societal change.
Centres and institutes
Our staff engage strongly with many of the research centres and institutes across the University.
Impact
Research throughout our Department is having a major impact in industry, society and beyond.
Facilities
Explore the range of top-class facilities that underpin all of our research.